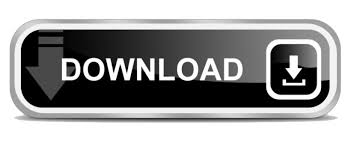
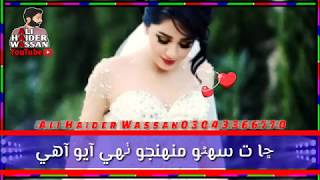
(c) HeLa cells were transfected with wild-type and 5′ splice site mutant NR3C1 (mutated from AAGGTAAGA to GTCCATTCA) mini-gene. (b) 3′ RACE was carried out as described in Figure 4a using RNA samples from HeLa cells transfected with control or U1 AMOs (7.5 μM) with or without SSA (100 ng/ml) for 8 hrs. (a) 3′ RACE was carried out as described in Figure 4a on the endogenous NR3C1 and STK17A genes using RNA samples from HeLa cells transfected with control, U1 (7.5 μM, 8 hrs) or U2 AMO (25 μM, 8 hrs), or treated with SSA (100 ng/ml, 8hrs). These findings reveal a critical splicing-independent function for U1 snRNP in protecting the transcriptome, which we propose explains its overabundance. We further show that U1 snRNA-pre-mRNA base pairing was required to suppress premature cleavage and polyadenylation from nearby cryptic polyadenylation signals located in introns.
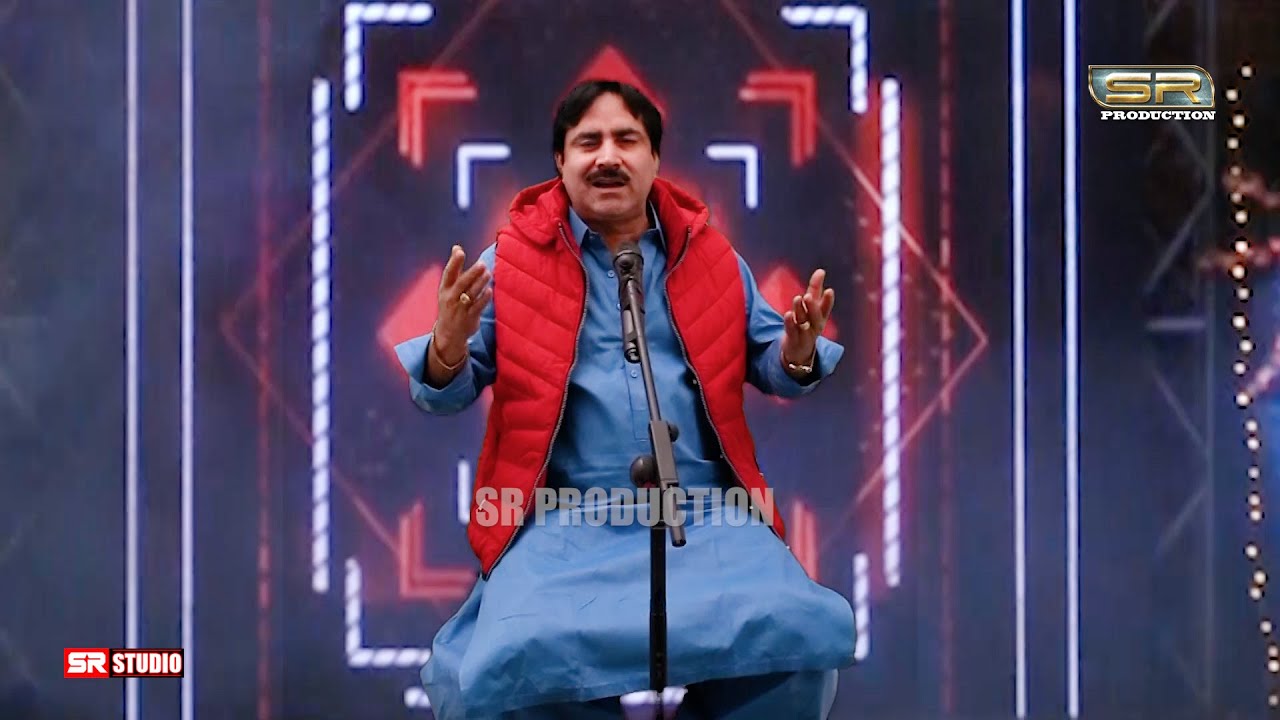
This did not occur when splicing was inhibited with U2 snRNA antisense morpholino oligonucleotide or the U2-snRNP-inactivating drug spliceostatin A unless U1 antisense morpholino oligonucleotide was also included. In addition to inhibiting splicing, U1 snRNP knockdown caused premature cleavage and polyadenylation in numerous pre-mRNAs at cryptic polyadenylation signals, frequently in introns near (<5 kilobases) the start of the transcript. Here we used antisense morpholino oligonucleotide to U1 snRNA to achieve functional U1 snRNP knockdown in HeLa cells, and identified accumulated unspliced pre-mRNAs by genomic tiling microarrays. doi: 10.1021/ eukaryotes, U1 small nuclear ribonucleoprotein (snRNP) forms spliceosomes in equal stoichiometry with U2, U4, U5 and U6 snRNPs however, its abundance in human far exceeds that of the other snRNPs. Phospholipase A2-Induced Degradation and Release from Lipid-Containing Polymersomes. Modification of Extracellular Vesicles by Fusion with Liposomes for the Design of Personalized Biogenic Drug Delivery Systems. Piffoux M, Silva AKA, Wilhelm C, Gazeau F, Tareste D. Cell-Templated Silica Microparticles with Supported Lipid Bilayers as Artificial Antigen-Presenting Cells for T Cell Activation. Olden BR, Perez CR, Wilson AL, Cardle II, Lin YS, Kaehr B, Gustafson JA, Jensen MC, Pun SH. A vesicle-to-sponge transition via the proliferation of membrane-linking pores in ω-3 polyunsaturated fatty acid-containing lipid assemblies. PubMed ID: 30977658Īngelina Angelova, Borislav Angelov, Vasil M. Effect of Formulation Method, Lipid Composition, and PEGylation on Vesicle Lamellarity: A Small-Angle Neutron Scattering Study. Nele V, Holme MN, Kauscher U, Thomas MR, Doutch JJ, Stevens MM. Anti-quenching NIR-II molecular fluorophores for in vivo high-contrast imaging and pH sensing. Wang S, Fan Y, Li D, Sun C, Lei Z, Lu L, Wang T, Zhang F. Increasing the penetration depth of temporal focusing multiphoton microscopy for neurobiological applications.
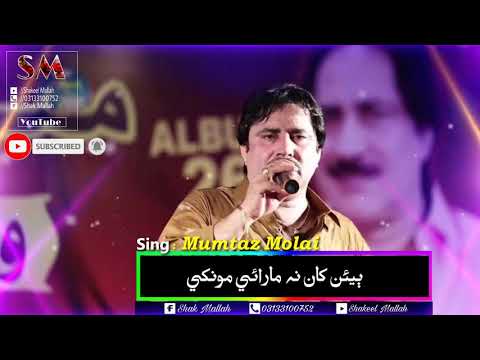
PubMed ID: 31218627Ĭhristopher J Rowlands, Oliver T Bruns, Daniel Franke, Dai Fukamura, Rakesh K Jain, Moungi G Bawendi, and Peter T C So. A Guide to Tracking Single Membrane Proteins and Their Interactions in Supported Lipid Bilayers. Single-molecule visualization reveals the damage search mechanism for the human NER protein XPC-RAD23B. PubMed ID: 31848341Ĭheon NY, Kim HS, Yeo JE, Schärer OD, Lee JY. Structural basis of nucleosome assembly by the Abo1 AAA+ ATPase histone chaperone. PubMed ID: 31942243Ĭho C, Jang J, Kang Y, Watanabe H, Uchihashi T, Kim SJ, Kato K, Lee JY, Song JJ. The structural fate of lipid nanoparticles in the extracellular matrix. Bandara SR, Molley TG, Kim H, Bharath PA, Kilian KA, Leal C.
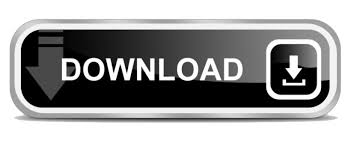